.jpg?auto=compress%2Cformat&crop=faces&fit=crop&fm=jpg&h=360&q=70&w=540
)
Humans are two developmental stages away from monkeys
Less than 50 of our 20,000 genes are unique to humans. What separates us from monkeys?
Anyone with access to a television can probably think of at least one movie comparing people to primates. There's MVP: Most Valuable Primate, or The Planet of the Apes. The trope in science-fiction books is even more popular. As these popular books and movies suggest, we seem to be forever asking the question—just how are we different?
At the most fundamental level, we're nearly identical to apes. Humans share 99 percent of our genetic information with chimpanzees, our closest relatives, and 93 percent with rhesus macaques, one of the most common monkey species. Out of our roughly 20,000 genes, less than 50 are exclusive to humans. From such tremendous similarity, how do our substantial differences arise?
The PsychENCODE Consortium—a U.S. government-funded initiative, involving 116 researchers at 15 institutes—is trying to finally come up with an answer. The collective recently published their findings as a series of 11 papers in a special issue of Science. One of these studies, spearheaded by Yale neuroscientist Nenad Sestan, radically redefined our understanding of how our brains become human.
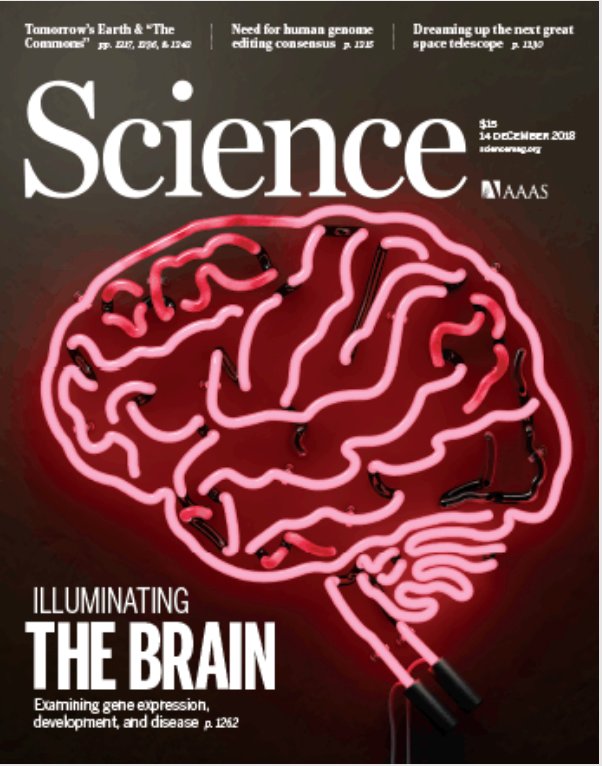
Science, AAAS
Sestan’s team studied both humans and rhesus monkeys as they grew up, from infancy into early adulthood. The researchers followed how the human and monkey brains changed over time, using a technique known as single cell RNA sequencing. This allowed them to isolate individual cells from the brains at different ages, profiling the amount of every gene in each cell. This means that the scientists could track changes, uncovering differences that are masked when millions of cells are combined, as they are in traditional genetic tests.
The amount of a gene in each cell can matter just as much, if not more, as the identities of the genes themselves. Consider baking cookies: Two recipes can have all the same ingredients, but if one calls for twice as much salt or baking powder, the final results will be dramatically different. If what separates people from primates is a result of how many genes of a certain type are in certain groups of cells—well, this would be the first study that could possibly detect these differences.
The researchers quickly noticed that for the most part, humans and monkeys don't actually diverge much as they're growing up. Humans go through every stage of macaque development, and most aspects of brain growth happen at the same time across the two species. In both humans and monkeys, brain cells—including neurons, cells that send messages to and from the brain, and glia, the cells that support neurons by providing nutrients and protection—develop and diversify in the same order, and at about the same time. As a result, our brains don't just grow in parallel—during certain periods, they actually become more similar to each other as they develop.
The next logical question, is what, exactly, causes humans to deviate so radically from monkeys? The Yale researchers found a few notable differences: Though humans advance through all stages of macaque development, humans also have two extra stages of brain development, ones found in no other animal. The first comes at the very beginning of life, when fetuses experience a short period of great change. Interestingly, during this phase fetuses show developments in their prefrontal cortex, which is crucial to complex thought and decision making, the most distinctive parts of human thought, and an area of the brain that's comparatively. After this period, our brains rapidly become more similar to those of monkeys—until the next period of divergence.
Sestan’s team found this second period comes during late childhood, around the age of ten. Humans have a prolonged childhood, meaning we take longer to mature, and also keep some childlike traits, like having large eyes and heads, permanently. But until now, scientists didn't understand why we take so long to develop. Only around the end of elementary school do the brain cells from our two species begin to form connections and transmit information differently. As a result, the researchers speculate that this phase could be responsible for how humans learn, think, and remember differently than monkeys.
Surprisingly, in all stages of development, humans and monkeys shared most cell types. This means if a neuron is present in humans, it is likely present in monkeys too. Our two species' genetic differences are generally found in a few small groups of cells, mostly subgroups of neurons. But even in these, the genetic differences across species remained relatively small—in total, the researchers found out of 20,000 genes, only about 50 diverged significantly. Despite the small number, some of these genes play major roles in mental function; many have been linked to disorders such as schizophrenia or bipolar disorder. For example, the gene GRIA1 controls learning and memory, causing schizophrenia by distorting the underlying basis of thought.
.jpg)
Prem Jejurkar on Pexels.com
Even though this work represents a major step forward in our understanding of what makes humans unique, this study only examined the genes found in a cell. Human and monkey brains may also differ in many other ways—like how brain cells connect to one another, or how these cells communicate. Further research along these lines will be necessary to fully understand the relationship between our two species.
Regardless, Sestan’s study illustrates how seemingly minor changes can yield major effects. Only two short periods of development meaningfully separate the brains of humans and monkeys, where only a few cell types receive the brunt of these changes. A minute number of genes diverge in just a few cells, and suddenly, we acquire the ability to read, to write, to speak—to be human.
After the Planet of the Apes came out, there was some speculation on what hypothetically could've made these apes become so close to humans, and what such a scenario would require in real life. We now finally have an answer: not much at all.
Fascinating story James. I just found the lack of distinction between gene and transcript very confusing, I had to keep reminding myself you meant RNA levels and not multiple copies of the gene. Also when you say “in total, the researchers found out of 20,000 genes, only about 50 diverged significantly” are you referring to the 55 genes they found differentially expressed in the single-nucleus RNAseq data? Because [as far as I know] they only detected about 450-2500 genes in each of those samples anyways, so the 20,000 number is incorrect.
Also, what do you think of this story about researchers claiming to have transferred a single human gene into macaques and found an increase in intelligence? Credible? And does that mean that they’re just fifty odd genes away from engineering human-like intelligence in monkeys?