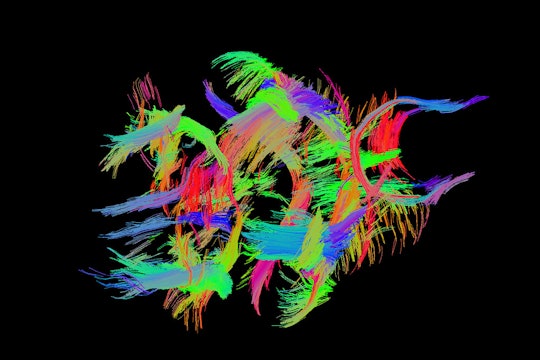
Susan Schwerin, M. Budde, M. Shindell, J. Munasinghe, S. Juliano, L.G. Cohen, National Institute on Neurological Disorders and Stroke, National Institutes of Health
Two mini microscopes watched a mouse’s brain move its body in real time
The NINscope will help researchers uncover how neurons in different regions of the brain interact with each other
Nearly every move we make, from binge-watching Netflix to debating whether to finally learn some baking, is controlled by the brain. Different parts of the brain have different functions, and we are still only a fraction of the way through to understanding what each of these parts do. The realization that parts of the brain work as a network forms the foundation of current neuroscience research.
To work out the networks that create specific behaviors, neuroscientists need live peeks into different parts of the brain, while also recording the actions performed by the animal attached to the brain they are peeking at. In a recent study published in eLife, a group of scientists from the Netherlands figured out how to simultaneously monitor two brain regions, using miniature microscopes, called NINscopes, that can be directly mounted on the head of a mouse.
The miniscopes are connected to a camera, letting researchers see what’s going on in the mouse’s brain.These devices are so small and light that not one, but two of them can be mounted on a single mouse. This nifty capability allows brain researchers do something they have been trying to achieve for years: observe two brain regions at the same time, while making sure that the mice they are studying are free to move around and behave normally.
Using two NINscopes at once, the scientists behind the new study were able to see neurons glow in two parts of the mouse brain – the cerebellum and the cortex – while the mouse was moving. A glowing neuron is an active neuron, so the researchers could match movements made by the mouse to specific patterns of neuronal activity. From these patterns, they figured out that the cerebellum and cortex work with each other to control some aspects of movement, but not all of them.
The ability to visualize neurons in freely moving mice has historically been a difficult feat to achieve. It is a tricky and delicate procedure even in immobilized live mice, requiring time and surgical finesse on the part of the scientist. Traditionally, this was done by sticking electrodes into the brain and recording the electrical signals generated by the neurons. While this technique gives precise readings of neuronal activity and timing, it does not allow researchers to see exactly which neurons are active. At best they can only assign activity to select regions, but not specific neurons.
In the 1990s, a new technique to record brain activity arrived, where scientists could use microscopes and special molecules called calcium indicators to observe neurons in action. When brain cells are activated, their interiors are flooded with calcium. The indicators bind to calcium and glow, making active neurons light up like fireflies. This effect can be captured very easily with a microscope and camera. What’s more, a single microscopic field can capture hundreds of cells at a time. Unlike the previous approach of using electrodes, calcium imaging lets scientists directly point out which neurons are active and when, just by looking at them.
Miniscopes also use calcium indicators, but they bypass a major shortcoming of traditional microscopes, which is that all imaging has to be done from a mouse that is restrained. While images from restrained mice are of good quality, you can only study a very small set of behaviors from such mice. But when using miniscopes, researchers no longer have to worry about restraining the mice. Once the miniscope is in place, the mouse is allowed some time to recover from the process and is then free to go about its daily routine. Miniscopes give researchers freedom to correlate a plethora of behaviors, ranging from sleep-wake cycles to social interactions, with brain activity.
The invention of the miniscope solved the issue of imaging brain cells in freely moving mice, but it still limited scientists to observing only one region at a time. When studying neuronal ensembles, it is important to visualize multiple brain regions in actively behaving mice.
This is where the NINscope offers a unique advantage over other miniscopes. It combines the dual-imaging capacity of traditional microscopes with the ability of miniscopes to track behaviors in unrestrained animals. The researchers custom-built electronic and optical components to build the NINscope, allowing them to shrink the instrument, house it in a 3D-printed shell, and place two NINscopes on a single mouse to study interactions between brain regions.
The NINscope does have some limitations. Its main selling point of dual-imaging, meaning simultaneously visualizing two brain regions, is not actually possible for any two regions of choice. The NINscope design mandates that a minimum distance has to be maintained between two mounted devices, meaning that there is some physical constraint on the combination of regions that can be imaged. It is also not clear whether the NINscope has any long-term effects on the mice. This is important to assess since experiments that analyze behaviors often run into weeks, in this case requiring the mice to go about all their usual activities with two miniscopes on their heads.
Nonetheless, the NINscope sets the stage for a large range of experiments aiming to map out potential neural networks. Weighing a mere 1.6 g (about the weight of 1.5 paperclips), the device comes power-packed with a few extra features. Researchers can use it to manipulate brain cells and see how they react using a module for optogenetic stimulation, which is a method where one can use light to artificially activate a neuron. With the proper modifications, they can also use it to look deeper into the brain. The NINscope also comes with a built-in accelerometer, allowing scientists to track the movements of the mice.
The NINscope is the culmination of years of smaller advances in technology. It is also a reflection of the multidisciplinary nature of science; the team needed expertise in the fields of optics and electronics to make the device, as well as in the field of neuroscience. An instrument like the NINscope offers an avenue into investigating neuronal ensembles, laying the groundwork for understanding how our brains function as a network.
Nice read! As you mentioned about the limited knowledge about the long-term effects of using NINScope, then how would they justify the data with long-term behavioural patterns in mice to check whether any significant effect of having the microscope attached is seen? And, you mentioned limitation of choices of brain regions, is it possible to study one region with NINscopes and other one with any other imaging methods?