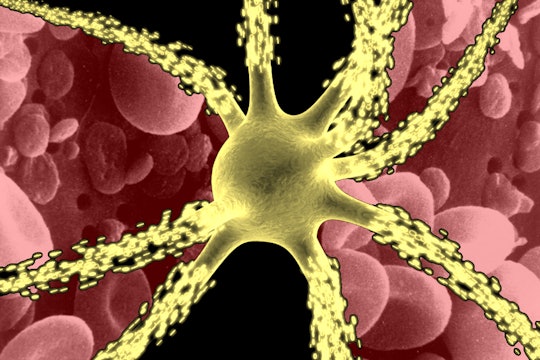
We're beginning to understand why spinal cord tissue is so hard to heal
A group of recent findings may eventually pave the way for better treatments
Every Wednesday night, my friends and I meet at a pub in the Plateau neighborhood of Montréal to play trivia. We are like a geekier, adult version of the Breakfast Club: the paleontologist, the librarian, the tour guide, the joker, and me – the neuroscientist.
Between rounds one recent week, I start telling my friends about a paper I read recently on glial scars, the type of scars that form after an injury to the central nervous system (CNS). Chris (the joker) tells me that this is a big problem when dealing with spinal cord injuries, because the scar makes a kind of wall that prevents the regrowth of injured pathways. Chris isn’t one of my scientist friends, but he knows a lot about this topic. He has a spinal cord injury.
And he was exactly right. Scientists consider getting around these ‘walls’ that glial scars build in damaged CNS tissue to be a major hurdle in treating injuries to the brain or spinal cord. But it turns out that this explanation doesn’t fully capture what is going on in the injured CNS.
Generally speaking, when the body is injured, a lot of things start happening. Almost immediately, platelets stick to the wound site to begin the clotting process, slowing blood loss. Inflammation sets in, bringing in white blood cells to eat away the dead and damaged cells. Finally, your body regrows tissue to cover the injured site. But what grows over the wound site isn’t exactly like the tissue it replaced – it’s a scar. And scar tissue has less of the stretchy connective fibers we call elastin, making it a lot stiffer than the surrounding tissue.
.jpg)
Scar tissue on an arm.
This is true for injuries to skin, to the heart, the lungs, or to other organs. And for a long time, scientists assumed that this was also true for the CNS – the brain and the spinal cord. But the CNS tends to do things a little differently than the rest of the body. Specialized cells called Glia coordinate the healing process in the CNS, which at a surface level looks a lot like the process elsewhere in the body: seal the injury site, get rid of dead cells, and rebuild the tissue. The end result is these glial scars, which we still don’t fully understand.
Scientists thought that these glial scars in the CNS made stiff physical barriers that prevent regeneration of damaged pathways and contribute to why these types of injury often lead to permanent damage, such as paralysis. But this idea was based off of what they knew about scars elsewhere in the body. Knowing more about the specific properties of glial scars and why they impede regeneration could help clinicians develop more effective therapies for treating injuries to the brain, so they set out to test what makes glial scars different from healthy CNS tissue.
However, it’s not a simple task to just test what glial scars are actually like. Consider this: first, scientists need to get into the brain and spinal cord. And then once they’re in there, they need to be able to measure very, very small differences in stiffness. On top of that, CNS tissue is already very soft and elastic – much more so than skin. So making measurements precise enough to get an accurate picture of how the elasticity of CNS tissue changes after injury is challenging.
A team of researchers from University College London, Cambridge University, and Brighton University recently addressed this challenge by using a technology called Atomic Force Microscopy (AFM). Whereas a traditional microscope extends the ability to see things, AFM extends the ability to feel things. So, this technique can be applied to 'feel' the tiny differences in elasticity in brain tissue. It works by seeing how much pressure the material needs to deform and then return to its original shape. A quantitative poke, if you will. Stiff materials need more pressure to deform in comparison to soft materials, so variations in the amount of pressure required tell how elastic the specimen is.
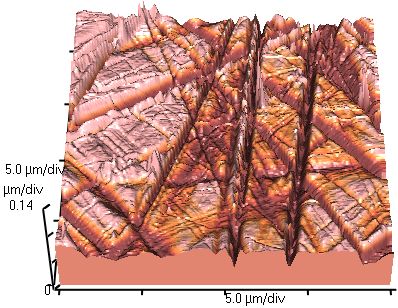
An example of Atomic Force Microscopy that shows the roughness of a plate of glass
Using this technique, scientists were able to measure the elasticity of rat brain and spinal cord tissue before and after injury. And what they found was the opposite of what everyone thought. Instead of glial scars in the CNS being stiffer than the surrounding tissue, like scars elsewhere in the body, they are actually softer.
But just because glial scars aren’t building a physical wall doesn’t mean that they aren’t a problem. In the healthy central nervous system, information is carried over long distances by axons: the long, thin projections of neurons. When the CNS is injured, these axons are often damaged or completely severed, and they generally do not regrow. Axons interpret chemical signals in their environment that tell them to stop or to keep growing, and a lot of research has been focused on manipulating these signals to encourage axons to regrow after an injury.
However, a mounting body of new evidence shows that axons also find their route by actually feeling the topography of their environment, and they don’t like to grow over softer surfaces like glial scars. Scientists are not sure why that is yet, but it could be the key to new, effective treatments to encourage axon regrowth by manipulating both chemical cues and tissue stiffness to create an environment that axons like to grow through.
While scientists are hopeful that this new information will spur the development of new therapies, they might still be far in the future. In the meantime, we still have work to do on the accessibility front: our weekly trivia night takes place in one of the few accessible pubs in Montréal.
This is a great illustration of how new technology can rejuvenate a longstanding debate. A lot of times in neuroscience, it feels like new microscopes are just used for their own sake (they are very cool). But here, using atomic force microscopy (AFM) may be the best way to test the hypothesis many people have been relying on, that gilal scars are actually “tougher” for axons to move through. If they aren’t, as suggested by AFM, then we need to be asking fundamentally different questions.
It’s also important, as we move from theories to therapies, to put actual numbers on things: how much force would an axon need to generate to move through a glial scar? If we knew, we would also know how far we need to go, rather than merely observing that we’re not there yet. Overall, this sounds like a great marriage of engineering and biology.