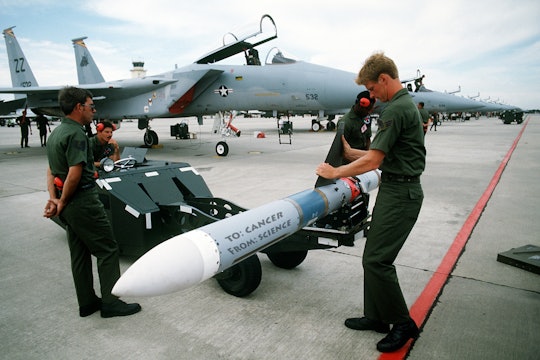
Modified from WikiMedia Commons
A chemotherapy missile made of DNA target-locks on to cancer cells
"Sticky" DNA sequences can guide drugs directly to cancer cells
DNA is the repository for genetic information. We've been tinkering with it since the 1970s, trying to alter the characteristics of organisms in a single generation, like boosting crop resistance to insect pests or even making pigs glow. Only recently have researchers popularized DNA as something else: a construction material. Earlier this year, researchers assembled an intelligent and autonomous nanostructure entirely out of DNA that delivered and released a cancer drug.
Cancer drugs are good at killing cancer cells, but they hurt healthy cells too. By concealing the drug, like a tiny sword, in a nano-sheath made from DNA, the researchers have made a secure, selective, and precise carrier for medication, thanks to DNA’s unique properties.
The war on cancer
Chemotherapy is one of the most common treatments for cancer, but it is notorious for its numerous possible side effects — hair loss, infertility, and a decrease in blood cell count, to name just a few. This is because anticancer drugs attack all rapidly dividing cells indiscriminately. While cancer cells fall into this category, so do certain healthy cells, such as those in the blood and in the lining of the gut.
To alleviate these side effects, anticancer drugs can be delivered with a carrier, one that masks the toxicity of the drug as the carrier complex traverses the body, ignoring healthy cells. Then, when it wanders into the vicinity of cancer cells, the anticancer drug is unleashed once and for all, to the detriment of only the cancer cells. Hopefully, the healthy cells won’t be caught in the crossfire.
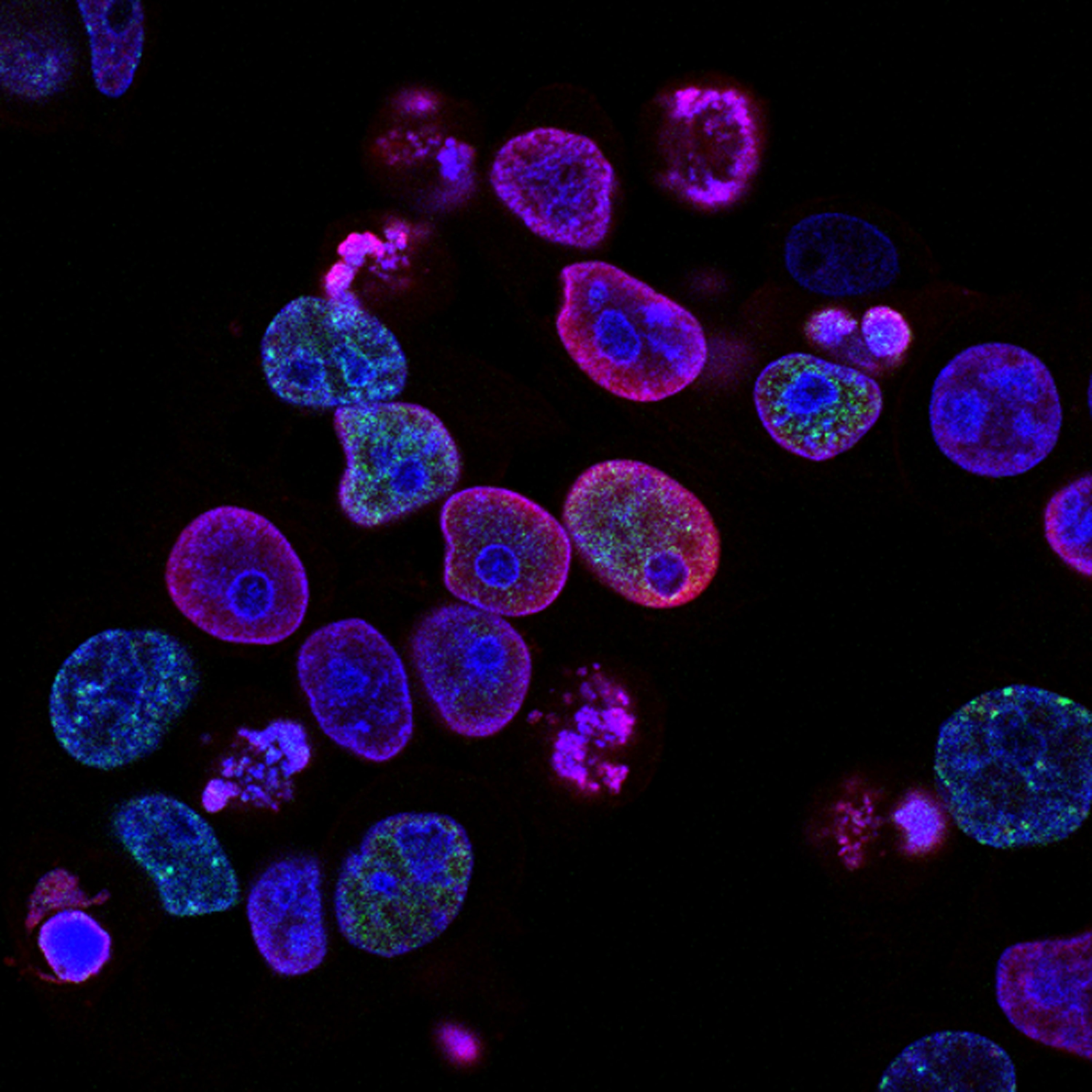
Human colorectal cancer treated with topoisomerase inhibitor
Photo by National Cancer Institute on Unsplash
An effective drug carrier needs to be selective in both the way it locates cancer cells and releases its toxic cargo. A handful of carriers work passively, seeping through the leakier walls of the cancer cells, as long as the carriers are sufficiently small. Others rely on a change in the local environment, such as temperature or ion concentration, to sense the presence of cancer cells. But in a highly variable environment like the human body, such strategies might not always be precise enough to distinguish the cancerous cells from the healthy ones.
This is where DNA comes in. It is a sticky but picky molecule. One strand of DNA is made up of a chain of different bases (the As, Gs, Cs, and Ts), and pairs with another DNA strand made from complementary bases (As stick to Ts, and Gs to Cs). Several studies have already exploited this selectivity for cellular recognition by DNA molecules.
As such, any drug carriers made from DNA strands will be highly specific in where they bind to. This is the basis for the "smart" DNA drug carrier design. The idea is to feed a patient, in advance, short individual strands of DNA (called aptamers) whose ends are known to anchor onto cancer cell surfaces. Then, the researchers administer the drug carrier that has been assembled from DNA strands complementary to the aptamers. The aptamers draw the DNA drug carrier to the cancer cells like a beacon.
Target engaged
“[Our drug carrier] acts as a DNA nanoscale precision-guided missile,” said Zai-Sheng Wu, one of the main authors of this study. Like a missile, the drug carrier molecule has a warhead and a guidance/control unit. The warhead is a DNA origami structure serving as the compartment for the drug. The guidance/control unit, another piece of DNA incorporated into the "missile," detects the aptamers on the surface of cancer cells, guides the carrier towards those cells, and unlocks the carrier's toxic contents based on a set of commands encoded in the DNA of the guidance/control unit.
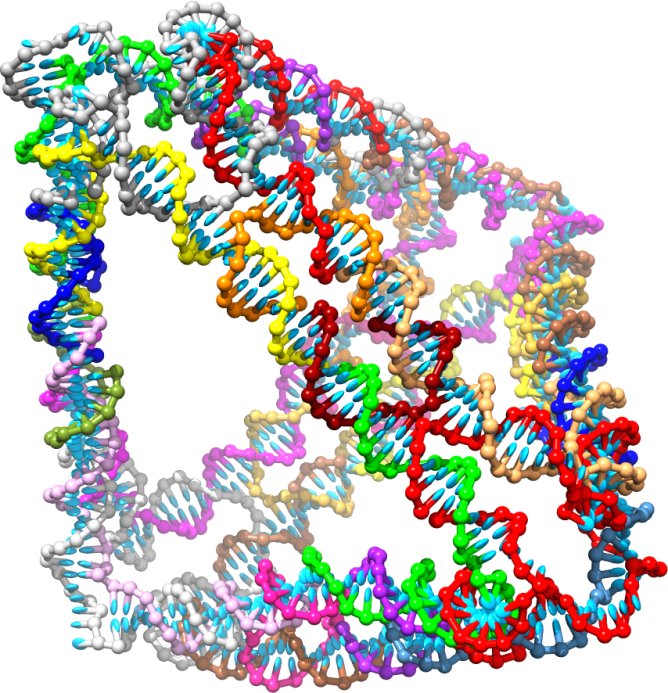
A DNA tetrahedron, a box made using DNA as a structure.
Professor Jonathan Doye, University of Oxford
An intriguing aspect of the carrier design is its unlocking mechanism, as executed by the guidance/control unit. Previous studies have similarly adorned their carrier molecules with DNA sensing abilities, but the drug can be taken up by any cell if the carrier loses its way or takes too long to arrive at its destination. This new missile-like drug carrier has an additional precaution built in — it requires three unique aptamer tags to activate the drug carrier, and they must do so in a particular sequence. Once the aptamers and the DNA nanocarrier stick to each other, the guidance/control unit disassembles and falls away, allowing the cancer cell to swallow up the carrier-drug. Like in a movie where it takes multiple keys to unlock a bomb's big red button, the drug only releases in the desired conditions.
Put to the test
To prove the efficacy of their DNA drug carrier, the researchers administered the aptamers and then the carrier into mice with implanted tumors. The DNA missile carrier had been loaded with doxorubicin, a common anticancer drug. After twelve days of repeated treatment, the tumors shrunk significantly, indicating a successful chemotherapeutic treatment. Meanwhile, the mice’s body weights had barely changed — a good sign of no ill effects.
This demonstration is promising for targeted drug delivery, acknowledged Amittha Wickrema, a cancer biologist at the University of Chicago who was not involved in the study. “This particular design seems [suited for carrying] doxorubicin,” he said. “Since doxorubicin is a widely used chemotherapy drug, [this work] will make a positive impact on the field [of cancer treatment].”
Wickrema said that more research needs to be done to understand the specifics of how the aptamers sticks to the cancer cells in the first place. Despite the bulwark of the guidance/control unlocking mechanism, it is still unknown whether the aptamers may glom on to cells other than on cancerous ones, which may falsely trigger the unlocking process. Until then, “The DNA drug carrier [should] not be the universal drug delivery system for cancer,” said Wickrema.
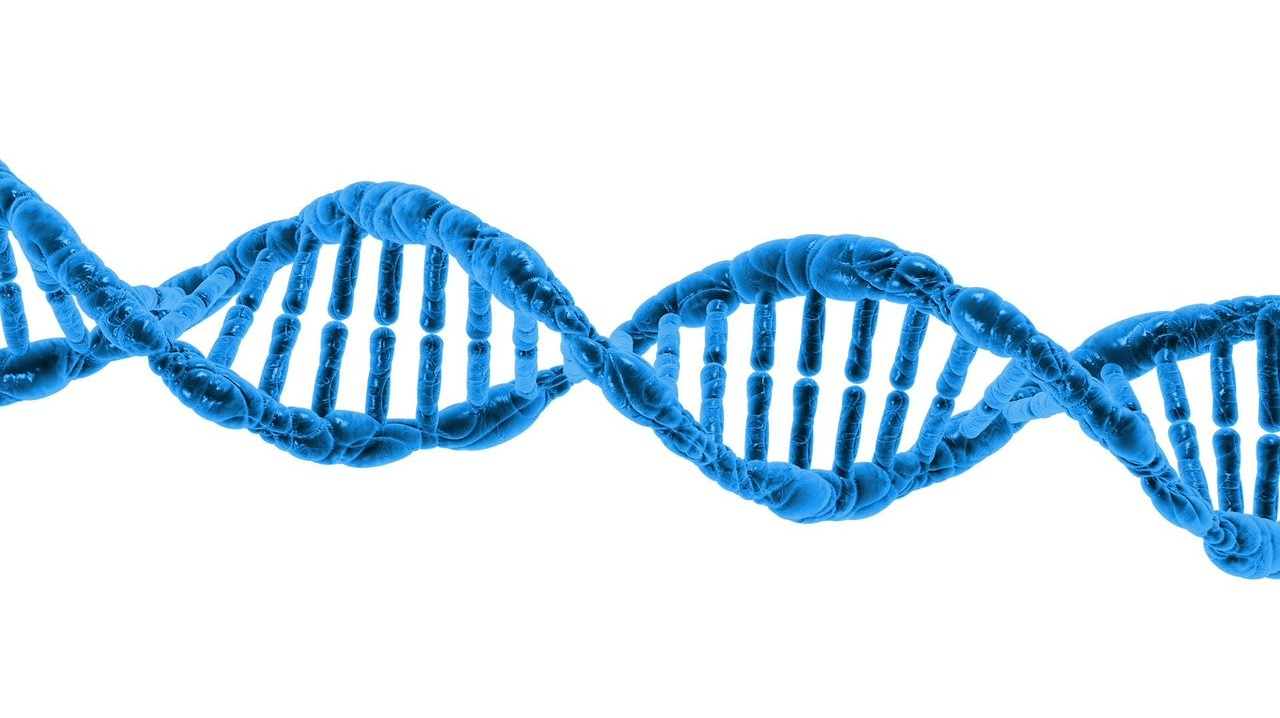
Researchers used DNA to encase a cancer drug, and guide the drug to its target.
DNA for nanotechnology
The rise of DNA as materials has opened up new opportunities at the nanoscale, ranging from molecular machines to autonomous origami. This research on the missile-like drug carrier is yet another instance of how DNA can be exploited for technological applications beyond Mother Nature’s intended purpose.
“DNA offers nanometer-scale control on positioning other materials,” says Ned Seeman, the scientist who founded the field of DNA nanotechnology in the early 1980s. The key lies in DNA’s sequence-determined stickiness: “[No other material platform] is so predictable.”
According to Seeman, there are about 500 labs worldwide that study the transformation of DNA strands into structural and functional materials. As a pioneer of the field, even he can’t predict where the field is headed towards — and that’s pretty exciting.
Perhaps missile-like drug carriers and glowing pigs are just the tip of the iceberg for what this versatile molecule has in store for us.
Nice article Kim. I do wonder why go to all the trouble to do this with DNA when there is another platform for targeted drug delivery already in use in the clinic: antibody-drug conjugates (ADCs), which take advantage of the precision of antibodies for binding a cancer cell-specific target epitope and delivering highly toxic cargo. ADCs are not perfect, one of their main issues is ‘leakage’ of the drug conjugate before reaching cancer cells, but many folks are taking aim at that problem with innovative solutions. I was disappointed that the original manuscript didn’t discuss the merit of DNA-drug conjugates compared to antibody-drug conjugates, but perhaps you have some thoughts on this?
As an aside, let’s not forget that Mother Nature itself has purposes for DNA beyond the genome. During an infection, immune cells called neutrophils shoot webs of DNA and inflammatory proteins (Neutrophil Extracellular Traps, or NETs), where the primary function of the DNA is to engulf pathogens while bombarding them with toxic inflammatory molecules.