Why there probably won't be a 'magic bullet' for cancer
Researchers increasingly view the disease as a sprawling, evolving metropolis of cells
In 1976, Peter Nowell put forth the idea that cancer was more than an overwhelming growth of cells. Just like a family tree, he argued, cancer is a collection of growing cells with diverse lineages and distinct characteristics. It was not until 2011, when we learned to sequence the genetic code of single cells, that scientists saw the vast, diverse cellular ecosystem of cancer. With the explosion of single-cell sequencing techniques since then, researchers are seeing, with unprecedented clarity, the varied behaviors of cancers and their evolutionary trajectories.
Cancer begins from a few mutated cells. As these cells develop, they mutate, grow, and experience different stresses from environmental forces. These forces influence the prevalence and survival of distinct cancer lineages, like a family tree for cells. Some cells may travel to other parts of the body, some may mutate and pass mutations on as they divide, and others may die due to treatment.
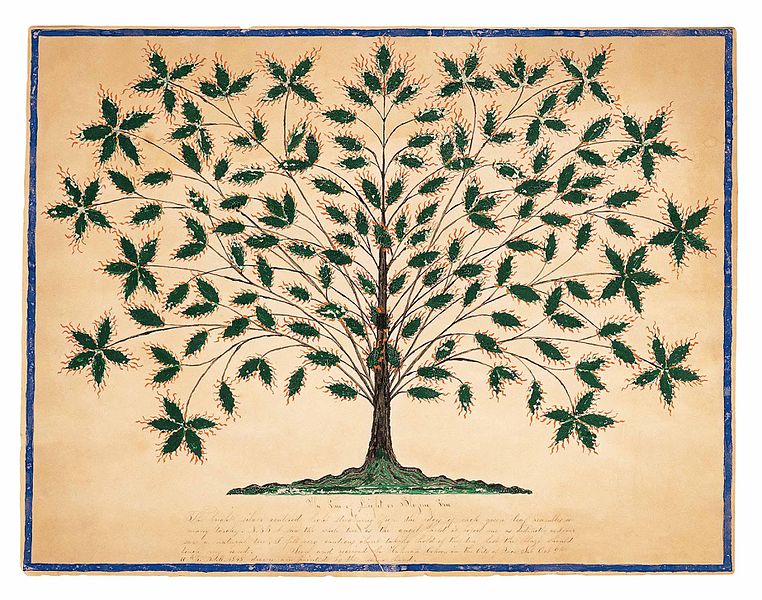
A metaphor
Currently, cancer treatments attempt to identify and kill these cells selectively, and normally ignore their evolution and diversity, though these factors have been implicated in why drugs develop resistance and treatments fail. For example, a patient may have a tumor comprising 99 percent of cells vulnerable to chemotherapy, and one percent of the cells that are resistant. Killing 99 percent of the cells with chemotherapy or immunotherapy may seem like a success, but what about the one percent left? These cells remain behind and grow into another diverse tumor, or travel to other areas of the body, causing remission. Although this diversity causes difficulties in the clinic, it also reveals the history of cancers, and shows potentially new ways to target cancer.
A 'Big Bang' theory of cancer
In an effort to comprehensively describe this diversity of cancer cells, researcher Andrea Sottoriva and his colleagues at Stanford University published, in 2015, what they call a “Big Bang” theory of cancer. Using colorectal cancer as their model, they showed a sprawling diversity among tumors. Since then, the field has seen a flurry of ideas to exploit this new view of cancer – a sprawling metropolis of distinct cells – including a study from earlier this year, by a team at the Francis Crick Institute in London, investigating the broad implications in cancer diversity.
This new view of cancer is changing how we think about diagnosing and treating it. In March 2016, researchers from the Koch Institute of Integrative Cancer Research at MIT, led by Boyang Zhao, investigated how they could take advantage of a concept called collateral sensitivity. Mostly studied in bacterial infections, collateral sensitivity is the idea that if a cell mutates in an advantageous way, it must also mutate in some disadvantageous way, a change called a fitness cost.
This fitness cost has been seen in the explosive invasion of cane toads in Australia due to rapidly evolving traits. Originally home to South and Central America, these toads have evolved longer legs and greater stamina in Australia, but at the cost of a weaker immune system. Similarly, if cells are mutating to confer resistance to a drug, maybe researchers can find a fitness cost to target during treatment.
According to the first computer models, these weaknesses can be acquired as the cells become resistant to current treatments, like chemotherapy. To identify this fitness cost within cancer cells, Boyang and his team treated acute lymphoblastic leukemia (ALL) cells with dastanib, a common therapy for blood cancers, and created more than 180 resistant cell lines. The researchers screened these cells against a panel of small molecule drugs, and found that multiple drugs, such as vandetanib, proved very effective. Digging deeper, they then investigated why this collateral sensitivity to other drugs, many of which did not share a similar traits as dastanib, arose.
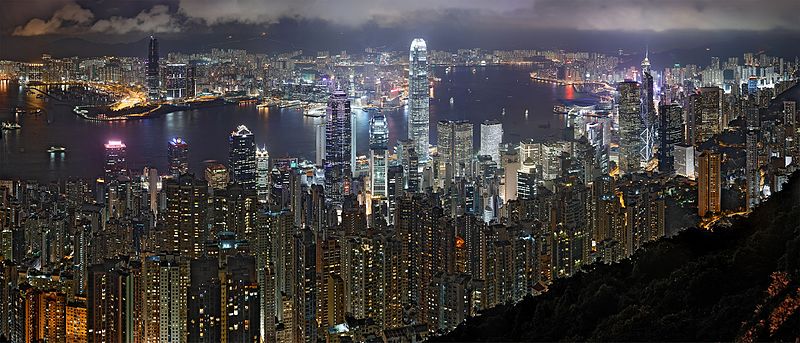
A second metaphor
Using genetic sequencing techniques, they found the culprit for why the cancer resisted one drug and became sensitive to another: a single amino acid change inside a protein. This amino acid change, from valine to lysine, increased the effectiveness of vandetanib to bind and affect a vital region of the target protein, ABL1. By modeling the growth, mutation, and death rates of these cells, Boyang's team predicted that this mutation made up approximately 0.008% of the initial cancerous population before treatment. Exposure to the original drug, dastanib, caused this specific mutation to grow and surpass other cells, helping a resistant cancer lineage gain a foothold during treatment.
Considering how to translate their work to the clinic, the team then checked their findings regarding vandetanib, among other successful drugs, with mice. In particular, they tried to find the best times to exploit the cancer's newfound sensitivity. By testing sequential drug combinations, they found changes in how the cancer cells developed or died. But ultimately, more work needs to be done to predict accurately how sensitivities arise during treatment, and how people might control them.
Boyang and his colleagues have demonstrated that, with the right research, we can learn how cancers evolve, exploit that evolution, and improve therapy in mice models. Still, more work is needed to identify how this rapid evolution of cancers can be captured and employed with different cancers and drugs. While there are many potential therapies, it's difficult to say how these ideas will – or won't – translate to the clinic. But given the known diversity of cancers and their evolving resistances, the strategies and concepts presented by Boyang show enormous potential: doctors could gain many more options for patients struggling with remission or failed treatment.
For instance, tests on the BCR-ABL1 gene sequence, responsible for the sensitivity identified by this work, are already commonplace for diagnosing in the clinic. But although the sequence itself is thoroughly studied, open research questions remain around when to test for it, and how to use our findings to guide treatment. With new understandings with how the sequence changes, in response to treatment, we could gain new value from these tests.
The missing 'silver bullet'
Over years of research, our original view of cancer, monochromatic and metastasizing, has transformed into a new vision: a complex city of cells, with varied traits and trajectories. As we continue to pick apart cancer in its many forms, we are increasingly seeing that a cure – a single "silver bullet" – likely won't be what many imagined. In fact, resisted and failed treatments show many parallels to the antibiotic resistance, itself a rapidly growing, diverse danger.
In both cases, we are dealing with versatile, dynamic organisms, and cancer poses an additional challenge: the organism is a supercharged, hyperactive part of our own body. Tackling cancer from this new ecological perspective could help scientists diagnose resistances before treatment, and then treat cancer effectively. New approaches would also mean changes to how we price and pay for new treatments: identifying distinct populations within cancer can help us predict effective treatments, before anyone doles out their savings for a six-figure therapy that may not succeed for the patient. Viewing cancer as this city of cells will help expand our toolbox for diagnosing and fighting the deadly disease, in all its motley legions.
This is a great piece, which breaks down the complexity of intra-tumor variability and presents it from an ecological perspective.
This personalized approach to drug resistance testing has already been used in treating HIV/AIDS by performing resistance testing, and successful treatment of HIV/AIDS is highly dependent on the appropriate management of drug resistances and combination anti-retroviral therapy.
The genome of a cancer cell is, of course, far more complex, and we need to obtain more knowledge about the genotype-phenotype-drug resistance correlates in cancer, making this a much harder endeavor.