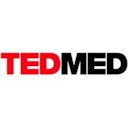
Produced in partnership with TEDMED
When your gut bacteria talk, your brain listens and replies
It's a conversation, one that may alter immune response and disease progression
The jury’s still out on how the brain really works. But Sarkis Mazmanian, a medical microbiologist at Caltech, thinks the answers to many of the questions we still have about the brain may actually lie further south — in the gut, where trillions of bacteria live. There, these “good” bacteria live peacefully, helping us to break down fiber and absorb nutrients. They are referred to collectively as the gut microbiome. Despite the presence of the blood-brain-barrier (BBB), a tightly regulated border between the brain and circulating blood, the gut and the brain are in constant communication, either through incoming and outgoing nerves, or through small molecules that can pass through the BBB. Remarkably, many of these molecules are not produced by the human body — they’re made by the bacteria in our microbiome.
The composition of our gut microbiome is often thought to be established as we pass through the birth canal, and greatly modified through our immediate environment in the first few years of life. After that, the microbiome becomes largely resistant to new bacteria. Interpreting this “gut-brain” axis has been the focus of Mazmanian’s work, revealing complex interactions between the gut and the brain, which increasingly look connected to everything from thoughts and emotions, to potentially the onset of certain brain disorders including Autism Spectrum Disorder and Parkinson’s disease.
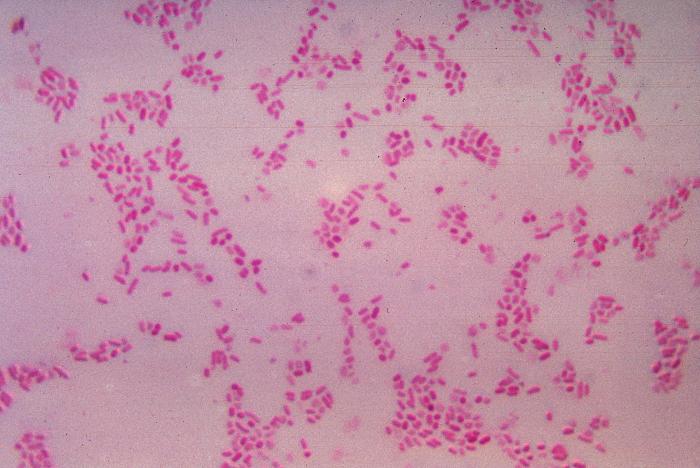
Bacteroides fragilis
CDC
You may be wondering a few things. How do trillions of bacteria establish themselves in our gut in the first place? How do our immune systems differentiate between the bacteria that make up our microbiome, and other harmful bacteria that makes us sick? Mazmanian says, “I think our microbiome, having evolved in the context of the immune system, have learnt to co-op with the immune system.” He adds, “Instead of trying to combat or invade the immune system, they actually engage it.” The good bacteria actually have a vested interest in their hosts being able to selectively attack dangerous bacteria, either because the “good” bacteria may also be harmed, either directly, or indirectly if their hosts perish. So, instead of avoiding immune cells, these beneficial bacteria have developed properties which redirects the immune response in a way that doesn’t cripple it. The “good” bacteria are spared, and the immune system is not prevented from attacking other pathogens. In this way, an amicable symbiosis is achieved, in which the gut microbiome is able to thrive in the warm, moist, nutrient-rich intestines. In fact, research carried out by graduate student Gregory Donaldson in Mazmanian’s lab suggests that one microbe in particular, called Bacteroides fragilis, might have even achieved long-term stability in the gut because of an immune response involving an antibody called IgA, which actually helps anchor it to the gut wall.
Mazmanian believes that our microbiome may influence many diseases. A few years ago, Mazmanian and his group noticed that children with autism — a neuropsychiatric disorder where children suffer from behavioral deficits, such as decreased vocalisation and social interaction, as well as repetitive behavior — also experience digestive issues, such as abdominal cramps and bloating. This was a clue that bacteria could be involved in the disease process. Other clues were that risk factors for autism include having a caesarean section, formula feeding, and taking antibiotics in childhood, all of which change the microbiome.
Mazmanian thinks the same may be true of Parkinson’s disease, a neurodegenerative disorder where neurons in the brain die, leading to motor symptoms like tremors, difficultly in walking, and rigidity. Like with autism, Parkinson’s patients often have gut symptoms. Strikingly, 80 percent of the three million people in the U.S. that suffer from Parkinson’s disease also suffer constipation—symptoms that sometimes precede the onset of motor symptoms. Interestingly, people who have had their vagus nerve, a potential highway between the gut and the brain, removed during surgery, are less likely to develop Parkinson’s disease.
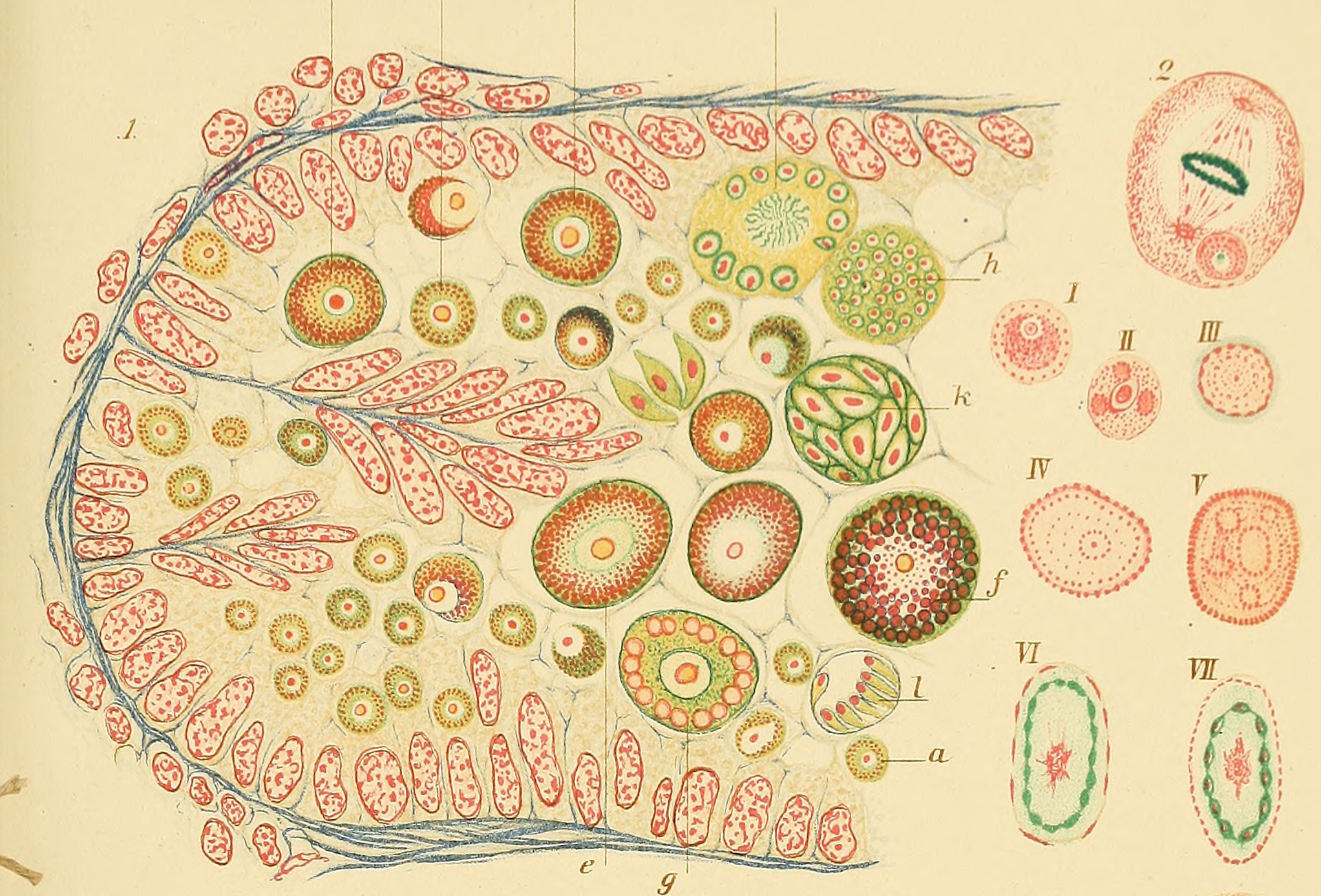
To study how the gut may influence neurological diseases, Mazmanian completely removed the gut microbiome of mice that are genetically engineered to develop autism or Parkinson's. He found these mice no longer exhibited symptoms of Parkinson's or autism, suggesting that the microbiome is involved in both diseases. Mazmanian had stumbled on a remarkable discovery. “When we made these germ-free sterile mice, it gave us a research tool that we can now use for other purposes.” Next, he took fecal samples (which contain intact microbiomes of their donors), from both Parkinson's patients and healthy controls. He put these samples into bacteria-free sterile mice genetically modified to over-express a protein called α-synuclein (αSyn, which is associated with Parkinson's disease). The mice implanted with microbiomes from people who had Parkinson's had much worse symptoms than the mice who received microbiomes from a healthy control. Similarly, when mice with autistic behaviors that had their microbiomes removed were given certain beneficial bacteria recovered from neurotypical humans , Mazmanian’s team were able to reduce their vocalisation deficits and repetitive behaviors.
Of course, these studies have only been carried out in mice, since there are ethical issues with replacing a healthy human’s microbiome with one from a Parkinson’s patient. However, Mazmanian says that dozens of papers have shown that the gut microbiome in autistic people and Parkinson’s patients are different. The cause of these differences — maybe ethnicity, geography, genetics or diet — is unclear, but Mazmanian’s mouse experiments have led him to a provocative hypothesis. He thinks some forms of autism and Parkinson’s may not arise in the brain at all, but in the gut. By targeting the microbiome, in a personalized way, he hopes to develop a viable therapeutic.
It’s not just the gut that sends signals to the brain. Weirdly, the brain also communicates with the gut, although understanding this process has been more challenging. Members of Mazmanian’s lab have been trying to better understand brain --> gut communication by working with neuroscientists using genetic engineering techniques, brain lesion studies, and studying the vagus nerve. Anecdotally, we rely on “gut-feelings” or “gut-instincts” to help us make decisions, sometimes we experience “gut-reactions” in response to an experience, and when we are overcome with anxiety or excitement, we often feel it in in our gut as a stomach-ache or “butterflies.” These turns of phrase suggest what these scientists suspect: that our brains send signals to our gut via our nervous system in response to queues in the environment.
Mazmanian’s lab are trying to not just identify the bacteria that inhabit our guts, but what these bacteria are doing. “We take a reductionist approach in the fact that we work with single organisms we can genetically manipulate,” he says. “I want to manipulate both the bacteria and the host,” isolating each on a molecular level to identify the mechanisms by which they work.
Conversely, Mazmanian likens many traditional drug treatments to pouring oil all over the engine of a car, in the hope that some might get into the right place. He thinks the future of medicine is in “drugs from bugs,” saying, “Someday, you and I may go to the doctor and be prescribed a pill with a live bacteria inside of it as the remedy.”