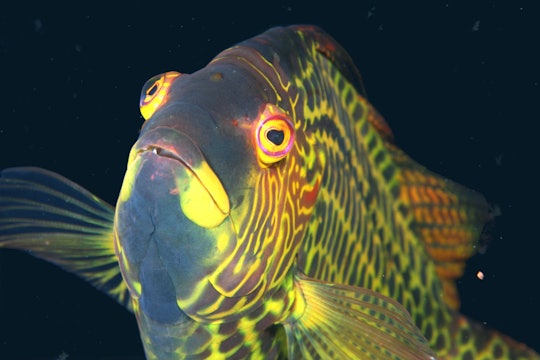
Klaus Stiefel / Flickr
What fish fins can teach us about how humans move
Studying fins could have surprising applications for medicine, engineering, and robotics
Have you ever tried standing on one foot with the other leg outstretched and your eyes closed? It’s tough to do without visual cues, but proprioception – the way appendages help the brain figure out where they are located in space – makes this possible. And more practically, injuries that damage the neurons that sense muscle stretching and transmit proprioceptive signals from limb to brain prevent you from being able to position your limbs correctly to make normal, effective movements.
This goes for animals, too. In 2015, Richard Williams and Melina Hale, University of Chicago scientists studying a combination of neurobiology and biomechanics, published their discovery that fish need proprioceptive sensation in their pectoral fins in order to swim effectively.
Their work and eventual publication intrigued Brett Aiello as he began his PhD work in Hale’s lab in 2012. As he settled in, he became interested in the universal importance of proprioceptive systems in the animal kingdom.
Yet, it was a different sort of biomechanical research that led Aiello to his PhD research project – my 2014 publication solidly focused on motor function, rather than sensation. In this study, my collaborators and I described an astonishing pattern we had stumbled upon while tracking animal movement in slow-motion YouTube videos: birds, bats, insects, fish, swimming mollusks, and marine mammals all bent their bodies in a very specific way as they moved. Material and evolutionary history didn’t matter. But why? And how? At the time, we proposed (and later I confirmed for fish) that the bending pattern was important for moving fast and efficiently through fluid environments – that is, swimming and flying well.
Wrasse flappers and rowers
Aiello was floored by this finding, as he shared when I met him in 2015, and began thinking about bending in fish pectoral fins, which are made up of a delicate membrane supported by long, flexible rays. If bending was important for swimming, and proprioception –specifically, sensing the bending in fin rays – was important for proper fin movement, were these systems linked?
To investigate, Aiello chose to work with a group of fishes called wrasses – brightly-colored coral reef fish that swim using their pectoral fins. Aiello already knew from collaborator Mark Westneat at the University of Chicago that wrasses swim in one of two ways, corresponding to the shape of their pectoral fins. First, a wrasse can be a flapper – using long, skinny, wing-like fins to “fly” through the water. Or, a wrasse can be a rower – using short, broad fins like oars to paddle.
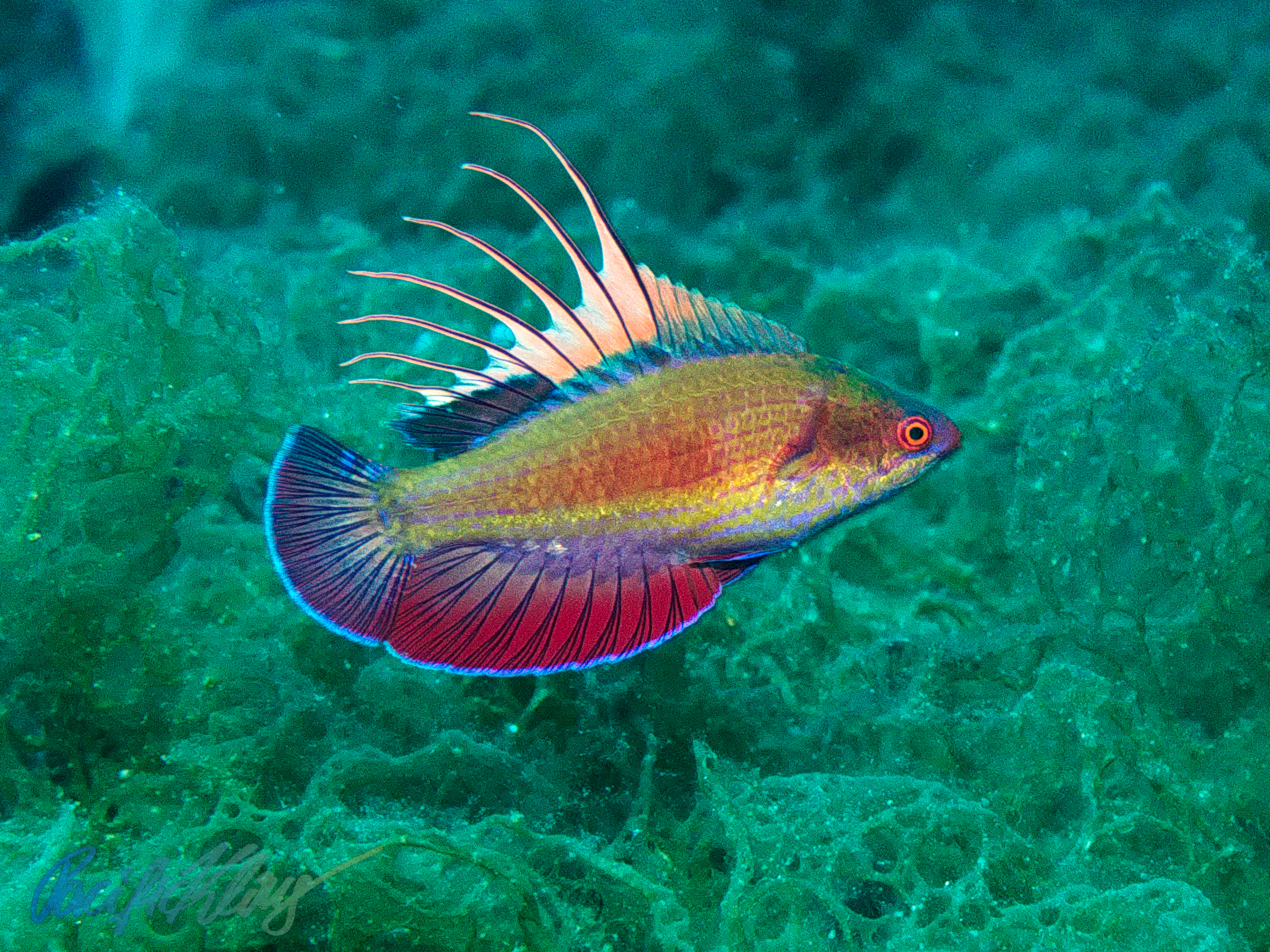
Wrasse
Klaus Stiefel / Flickr
From watching wrasses swim at aquariums and the like, Aiello also had noticed that the fins in flapping wrasse bend less than the fins of rowers. And, from Williams’ and Hale’s earlier proprioception work, he knew that fin rays can detect bending. Because sensory neurons respond to very specific cues, but factors like how a limb is moved and its stiffness vary, one type of sensor wouldn’t be useful in all limbs. Putting these pieces together, Aiello hypothesized that flappers would have stiff fins that would bend less during movement, and so their proprioceptive neurons would have to be more sensitive to small bends than the neurons in the more flexible rowing fins.
To test his hypothesis, Aiello needed to start by showing that flappers did indeed have stiffer fins than rowers. He picked out a pair of species – one rower and one flapper – that were closely related so that any differences he saw between species would be due to the difference in fin use rather than some accident of evolution. Aiello dissected out fin rays from each fish. Using a materials testing machine, a device from the engineering world that applies very precise force to a material and tracks how the material bends or twists in response, Aiello could measure the stiffness of each ray. Because he needed more force to bend a flapper fin ray the same amount as a rower fin ray, he was able to confirm that the flapper had stiffer fins.
Aiello then needed to see how sensitive proprioceptors were in each fin type. He placed electrically sensitive recording devices near the proprioceptive neurons of fin rays in intact pectoral fins. Aiello then carefully bent the rays and measured how the electrical signals produced by proprioceptive neurons changed. When he compared the results across species again, he found that the rower’s flexible rays could bend three times as far as the flapper’s stiffer rays before the proprioceptive neurons reacted with a large burst of electricity. This confirmed that a flapping wrasse’s fins were more sensitive than the rowing wrasse’s.
A wider view
Tantalizing as these results were, Aiello wasn’t satisfied. Sure, certain sensory responses, fin motions, and fin stiffnesses all fell into a distinct pattern in these two fish species, but there are millions of fish species out there. Unless he looked more broadly, how could he know if he had found a big trend, or merely a fluke?
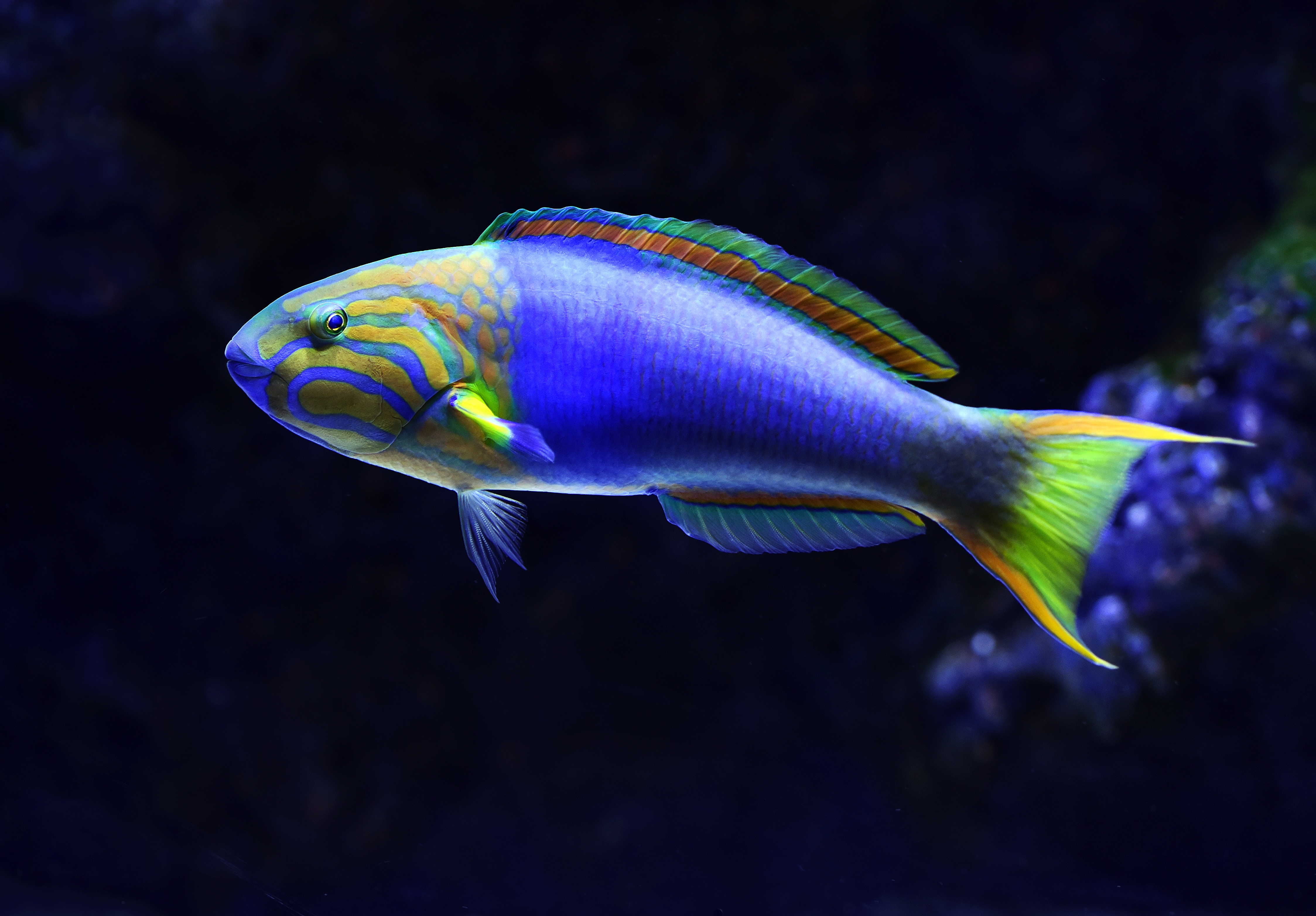
Of course, it's unrealistic to test every single species of fish. So, Aiello used DNA from 340 different wrasse species and a series of statistical tests to construct a wrasse family tree, where all the species were the “children” at the bottom of the tree, connected back in time by unknown ancestors until he reached one “granddaddy of all wrasses” at the root. Aiello then mined FishBase, an online database of fish facts, for pictures of wrasse fins, and labeled each of the 340 species as a rower or flapper. Tree and assignments in hand, Aiello could run another set of statistical tests to find out the odds of each ancestor being a rower or flapper.
Based on his calculated odds, Aiello predicted that the first ancestor of all wrasses had the fins of a rower. This meant that flapping had evolved out of rowing, and surprisingly, flapping evolved in 22 separate places on the tree: there wasn’t a particular flapper lineage from whence all flapping wrasse came. Rather, flappers were mixed among the rowers. When he used his tree to find three other closely related species pairs of rower and flapper, and repeated his earlier tests, he obtained the same results. It didn’t matter who the ancestors were; the sensitivity of the proprioceptive neurons always matched fin shape, movement type, and ray stiffness.
Because this happened so repeatedly, Aiello said at a recent conference, we could predict that this coupling may be a general principle in the evolution of neuromechanical systems.Through findings like Aiello’s, which were made possible by uniting the disparate fields of solid mechanics, phylogenetics, and neuroscience, biomechanists seek to understand how the systems we see in the animals around us – and in our own bodies – came to be. While this could help us understand the limits of our bodies, and why injuries can cause the trouble they do, these sorts of findings can help us overcome these limits. At the same conference where I caught up with Aiello, I also saw threads of his work spilling into engineering design, where a fish-fin-inspired propulsion system was detecting bending to allow the robotic controller to know where the fin was in space.
In the coming decades, we could see fish-like robotic vehicles helping us to understand the seafloor or to assess damage and find survivors from massive floods like those in Florida and Texas this past hurricane season. Or perhaps we’ll use further studies of fish proprioception to fully understand how these systems relate to our movements – and how we can better help injured humans move freely again. As we continue to cross disciplinary boundaries and build upon basic principles, I am excited to see what the future holds.